You can find details about how to access information remotely in this step-by-step guide. The guide will also help if for any reason you have difficulty accessing the content you want.
What would you like to know about this journal?
Impact factor: 4.2*
Time to first decision (all decisions): 21.0 days**
Time to first decision (peer reviewed only): 40.0 days***
Chair: Hiroaki Suga
Indexed in the Directory of Open Access Journals (DOAJ), PubMed Central, Scopus and Web of Science: Emerging Sources Citation Index
Where agenda-setting research has global visibility
Led by Hiroaki Suga, RSC Chemical Biology is a journal dedicated to publishing significant findings from the broadly understood chemical biology community. It provides a publication platform for communities of chemists who are developing chemical tools to resolve biological questions and biologists who explore the mechanisms and manipulate biological processes at the molecular level. The journal is gold open access so there are no barriers to accessing content and the research reaches a truly international audience.
“I’m very excited to take on the role of Chair of the Editorial Board of RSC Chemical Biology. This new journal publishes important research sparked at the interface of chemistry and biology. I’d encourage colleagues to submit their high quality work from basic to translational research to RSC Chemical Biology.”
Hiroaki Suga, Editorial Board Chair, RSC Chemical Biology.
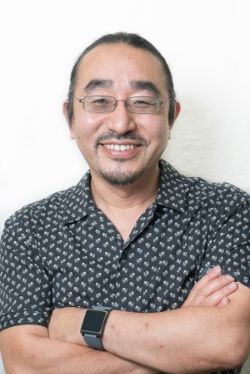
Cutting-edge
At the forefront of agenda-setting research in chemical biology, with an innovative, transparent peer review process
Visible
A gold open access journal, giving your work maximum visibility and reaching a global readership. APCs are waived until mid-2022
Trusted
Led by an editorial board of leading scientists, and published by a society committed to the wide dissemination of quality knowledge
Explore RSC Chemical Biology
Led by Hiroaki Suga, this journal is dedicated to publishing significant findings from the broadly understood chemical biology community.
Journal scope
RSC Chemical Biology is a gold open access journal dedicated to publishing and disseminating exceptional, breakthrough research and high-quality reviews at the interface of chemistry and biology.
We welcome contributions from across the breadth of the chemical biology field. This includes research on the development of new chemical and biological techniques and tools that comprise but are not limited to:
- Analytical methods (including sensing, imaging, spectroscopy, omics)
- Bioorthogonal chemistry
- Biosynthesis, biomimetics and bioengineering
- Chemical synthesis and synthetic biology
- Directed evolution
- Drugs and small molecules (including prodrugs)
- Glycoscience
- Lipids
- Natural products
- Nucleic acids (including DNA, RNA, mRNA and siRNA, modifications and gene delivery)
- Peptides
- Phenotypic screening
- Proteins (including protein-protein interactions, modifications, structure and function)
We are particularly interested in reports on the application of chemical tools to probe, explore and visualize biological systems and processes to provide insights into molecular mechanisms in health and disease. These include but are not limited to:
- biochemistry, metabolism, and physiology
- cancer biology
- immunology and immunomodulation
- neuromodulation
- transcription/gene regulation
- mechanism of disease and therapeutics
- signalling pathways
- virology and microbiology
- natural product biosynthesis
- systems biology
We also encourage translational research that bridges chemistry and chemical biology to medicine.
Computational studies must significantly advance chemical biology. Studies using established methods should present original predictions, validated by new experimental data. Novel computational methodologies must demonstrate relevance through direct comparison with experimental results. Studies lacking clear experimental connections or practical utility that meaningfully advances the field are unsuitable for RSC Chemical Biology.
Oustanding Early Career Researcher Award
We are deligthed to introduce the Outstanding Early Career Research Award 2023. This award aims to acknowledge and celebrate exceptional contributions made by early career researchers within the RSC Chemical Biology field. It serves as a means of recognition for their dedication, innovation, and impactful research endeavours.
Find out more about the award and meet the winners.
Transparent peer review policy
To support increased transparency, we offer authors the option to publish the peer review history alongside their article. Reviewers are anonymous unless they choose to sign their report.
Find out more about our transparent peer review policy.
See who's on the team
Find out who is on the editorial and advisory boards for the RSC Chemical Biology journal.
Editorial Board Chair The University of Tokyo, Japan
Associate Editor University of Würzburg, Germany
Associate Editor Nuffield Department of Medicine, Oxford University, England
Associate Editor University of Michigan, Ann Arbor, USA
Associate Editor University of Münster, Germany
Associate Editor Technical University of Berlin, Germany
Associate Editor Leiden University, Netherlands,
Associate Editor Shanghai Institute of Materia Medica, China
Editorial board member
University of California, San Francisco, USA
Editorial board member
Washington University, USA
Editorial board member
University of Southampton, UK
Luc Brunsveld, Eindhoven University of Technology, The Netherlands
Christopher Chang, University of California, Berkeley, USA
Peng Chen, Peking University, China
Benjamin Davis, University of Oxford, UK
Paul Dyson, EPFL, Switzerland
Dorothea Fiedler, FMP Berlin, Germany
Haian Fu, Emory University, USA
Christian Hackenberger, FMP Berlin, Germany
Don Hilvert, ETH Zürich, Switzerland
Guifang Jia, Peking University, China
May Khanna, New York University, USA
Laura Kiessling, MIT, USA
Maja Köhn, University of Freiburg, Germany
Yamuna Krishnan, University of Chicago, USA
Lei Liu, Tsinghua University, China
Chudi Ndubaku, ORIC Pharmaceuticals, USA
Hermen Overkleeft, Leiden University, The Netherlands
Jennifer Prescher, University of California, Irvine, USA
Christopher Schofield, University of Oxford, UK
Pamela Silver, Harvard Medical School, USA
Giulio Superti-Furga, Medical University of Vienna, Austria
Xiu-Jie Wang, CAS, China
Kira Weissman, University of Lorraine, France
Jin Zhang, University of California San Diego, USA
Xiang Zhou, Wuhan University, China
Anna Rulka, Executive Editor ORCID 0000-0002-3236-9801
Audra Taylor, Deputy Editor
Viktoria Titmus, Editorial Production Manager
Angelica-Jane Onyekwere, Assistant Editor
Zareena Saleem, Assistant Editor
Michael Whitelaw, Assistant Editor
Alexander Whiteside, Assistant Editor ORCID 0000-0002-1743-1531
Samantha Campos, Editorial Assistant
Jasmin AP, Publishing Assistant
Neil Hammond, Publisher ORCID: 0000-0001-6390-8874
Open access
We offer RSC Chemical Biology authors a choice of two Creative Commons licences: CC BY or CC BY NC. Publication under these licences means that authors retain copyright of their article, but users are allowed to read, download, copy, distribute, print, search, or link to the full texts of articles, or use them for any other lawful purpose, without asking prior permission from the publisher or the author. Read our open access statement for further information.
All published articles are deposited with LOCKSS, CLOCKSS, Portico and the British Library for archiving.
Dive into the benefits of open access publishing
Find out more about open access publishing routes
Explore Royal Society of Chemistry open access journals
Read our Researchers’ voice report in response to Plan S
Article processing charges
Article processing charges will apply as below to all articles submitted to RSC Chemical Biology if, following peer review, they are accepted for publication.
Article processing charge | |
---|---|
Full price | £2,100 (+local taxes if applicable)* |
Corresponding authors from India, Indonesia and Philippines | £1,050 (+local taxes if applicable) |
Corresponding authors from Research4Life Group A & Group B | Full APC waiver |
*15% RSC member and RSC open access agreement discount available. Applicable to full price only.
Use our journal finder to check if your APC is covered by an institutional agreement with us. Note, if your institution has an agreement with us that covers APCs, you will be notified of this at article acceptance.
Discounts and waivers are also available on an individual basis. Find out more about applying for a waiver.
Corresponding authors who are not already members of the Royal Society of Chemistry are entitled to one year’s Affiliate membership as part of their APC. Find out more about our member benefits.
As part of the submission process, authors will be asked to agree to the RSC Chemical Biology open access terms & conditions.
We offer RSC Chemical Biology authors a choice of two Creative Commons licenses: CC BY or CC BY NC. Publication under these licenses means that authors retain copyright of their article, but allows users to read, download, copy, distribute, print, search, or link to the full texts of articles, or use them for any other lawful purpose, without asking prior permission from the publisher or the author. Read our open access statement for further information.
All published articles are deposited with LOCKSS, CLOCKSS, Portico and the British Library for archiving.
For information about APC pricing for all RSC journals please click here.
Peer Review and Editorial Process
RSC Chemical Biology strives for a rigorous, fair and rapid peer review procedure. On submission to the journal, manuscripts undergo an initial assessment by a team of professional editors to ensure they meet essential criteria for publication in the journal; those manuscripts which are successful in this review are passed to an associate editor for further consideration.
RSC Chemical Biology follows a single-anonymised peer review process, with manuscripts handled by a team of internationally recognised associate editors, all practising scientists in the field. It consists of the following stages:
- Phase 1: Your manuscript is initially assessed by an associate editor.
- Phase 2: The associate editor solicits the expertise of at least two anonymous reviewers to assess your article and submit a report.
- Phase 3: The associate editor handling your manuscript makes a decision based on the reviewer reports received. In the event that no clear decision can be made, another reviewer will be consulted.
Please refer to our processes and policies for full details including our appeals procedure.
Subscription information
RSC Chemical Biology is fully gold open access – articles can be downloaded free from the website with no barriers to access.
Online only: ISSN 2633-0679
Copyright
Copyright is retained by authors when an open access licence is accepted, as with our standard licence to publish agreement. Full and accurate attribution to the original author is required for any re-use of the work. Find out more about copyright, licences and re-use permission.
Sign up to hear more from RSC Chemical Biology
Complete the form below to receive news alerts and issue alerts from RSC Chemical Biology.
*2023 Journal Citation Reports (Clarivate Analytics, 2024)
**The median time from submission to first decision including manuscripts rejected without peer review from the previous calendar year
***The median time from submission to first decision for peer-reviewed manuscripts from the previous calendar year
Pre-submission queries
- Email:
- Anna Rulka, Executive Editor